Discovery rewrites paradigm on genetic transcription
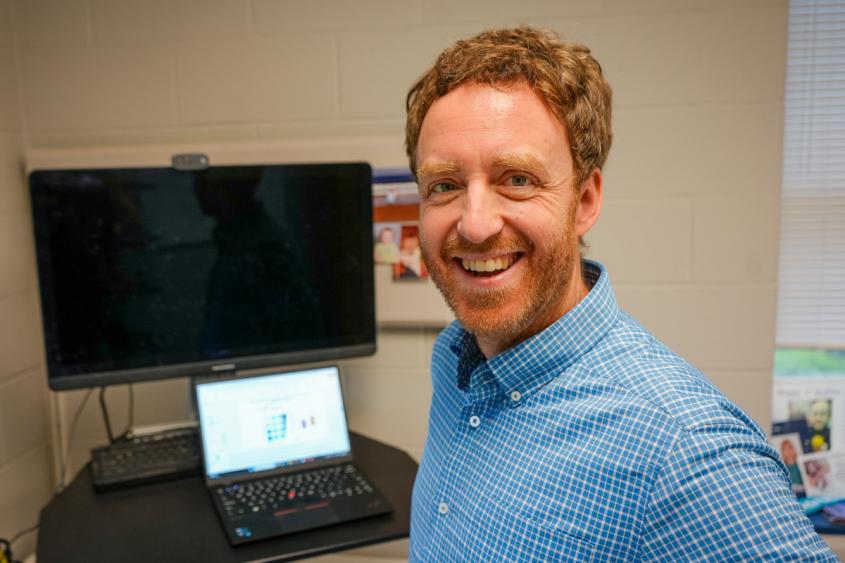
Researchers at the Cornell University College of Veterinary Medicine proved that gene enhancer-promoter interactions both drive, and are driven by, transcription, potentially discovering a new function for the transient pausing of RNA polymerases.
In a paper published in Nature Genetics, Dr. Charles Danko, Associate Professor at the Baker Institute for Animal Health, and his colleagues solved a long-lasting scientific debate about the interaction of two genomic elements, called enhancers and promoters, that interact to control gene expression. How our genes are expressed, i.e., turned on and off, affect many of our traits, including disease risk.
Promoters generally sit close to the gene they regulate, but enhancers can be located far away on the DNA strand. Enhancers regulate the timing or intensity of expression of a target gene by interacting with its promoter. One widely accepted theory of how enhancers and promoters interact is the looping model. In this model, the DNA bends to form a loop that brings an enhancer close to or in contact with a promoter.
However, some observations called this model into question. Microscopy images taken while genes are expressed clearly show the promoters and enhancers far away from each other. These microscopy observations suggested that enhancers and promoters did not need close proximity or contact to interact.
“It takes a lot of mental gymnastics to reconcile these studies,” says Danko. “There were many moments of sketching ideas on the white board.” When Danko and his team started their project, there were two hotly debated questions in the scientific community: First, how close are the promoter and enhancer during gene expression, and second, does a physical bridge form when these two genomic elements interact.
Initially, Danko was not trying to settle the debate, he only wanted to measure how frequently enhancers and promoters are close together. “Like many times in science, the way you get to a result is not how you started,” says Danko.
Believing that the contradictions were in part due to a resolution issue, the researchers used a different technology that allowed them to focus on tiny regions of the genome — a few hundred nucleotides — just the size of the elements they were looking at.
In previous studies, researchers used a technique called Hi-C where a series of chemical reactions freezes a living nucleus at one point in time, preserving DNA conformation with its loops and its points of contact. The immobilized DNA is then cut in fragments while maintaining the bonds between pieces that were in contact immediately before the chemical freeze. With Hi-C, the fragments can be quite large and encompass large stretches of DNA sequence around promotors and enhancers. “Looking at larger chunks can create all sorts of artifacts,” says Dr. Gilad Barshad, the first author of the study. These artifacts could explain the inconsistencies between the looping model and microscopy observation.
Danko’s team used a refined version of Hi-C called micro-C where the DNA fragments are much smaller, allowing the researchers to focus on enhancer and promotor sequences alone. They ran their experiments on well-studied cell lines, where they knew which enhancer-promotor pairs should be interacting with each other. Using this approach, their experiments unequivocally showed that interacting pairs spend more time close together.
“Basically, that shows that we cannot throw away the old looping model, and these results are not consistent with the microscopy observations,” says Danko. “But if frequency and proximity are important, then what’s the glue?”
The glue
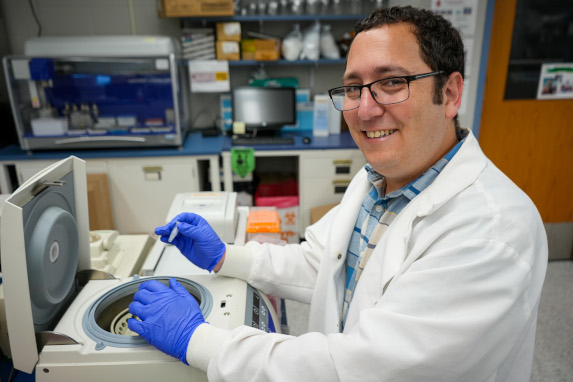
Looking for what brings enhancers and promoters close and holds them together, Danko and his team disproved existing assumptions one more time. They found that the same proteins that transcribe the genes also act as glue.
When transcription occurs, RNA Polymerase II, or Pol II, and other proteins come together to read and copy a gene into a messenger RNA. The idea that this transcription machinery could be the glue had been proposed before but did not get much support from the scientific community because experiments that removed one of the machinery components showed no effect on genome looping and interactions. But again, these studies relied on contact maps generated with Hi-C and did not have the resolution to focus on the enhancer-promotor interaction specifically.
“This brought the field to believe that transcription is a one-way street. Folding of the genome affects the transcription, but transcription does not affect folding,” says Barshad.
Using the higher-resolution micro-C technology, Danko’s team observed that removing one of the proteins of the transcription machinery did in fact change the enhancer-promoter contacts. This finding was momentous for the team. “When we realized the two-way street, it was real point of excitement,” says Barshad. “I thought we are onto something that is very important, and we should make it known to other people that transcription matters.”
Almost in parallel to this study, two independent teams, also using the high-resolution technology, published supporting observations. One of them removing Pol II, and one removing another protein of the transcription machinery, also showed that enhancer-promoter interactions rely on the transcription machinery, and Pol II in particular.
“It’s a watershed moment and now the community accepts that transcription affects genome folding,” says Danko.
A matter of state
Based on their observations, Danko and his team proposed a hypothesis for what happens at the transcription site. Scientists had previously established that when the transcription starts, Pol II starts moving along the DNA strand, and after reading thirty to sixty nucleotides, it stops. After this pause, which can last up to ten minutes, Pol II resumes its path along the gene.
Previous studies had shown that Pol II, among other proteins, plays a key role in enhancer–promoter communication. Going further, Danko and his team observed that in transcriptionally active genes, removing the pause specifically, without depleting Pol II, decreases the contacts between enhancers and promoters.
By using cell lines in which they could add or remove factors that pause Pol II, they could demonstrate that the presence of Pol II in its paused state is crucial for DNA folding and for enhancer-promoter interactions to happen. “Basically, the pause is the glue,” says Danko. “As a PI, you never believe the answer at first,” says Danko. “But at some point, you think, ‘Hey, this is real.’ ”
As a next step, Danko wants to know what other proteins are important for the ‘glue’. “We have preliminary data that shows that other proteins are involved in building a compartment where transcription can take place,” says Danko.
Ultimately, the more scientists understand transcription, the better they can understand its variations and their effects on our health. For Barshad, he wants to understand the link between some traits, like juvenile arthritis, and genetic variation in the enhancer DNA sequence. “I’m curious to know if that variation affects how active the enhancer is, or maybe how it can reach the promoter,” says Barshad. “To think of the next step, that’s what keeps me moving.”
Written by Elodie Smith